This site requires a lot of work. We hope you find our efforts valuable and rewarding. Please consider offering your support. There is no minimum amount. Feel free to donate as you see fit, without restriction. Thank you...
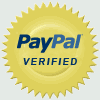
The Uranium Explosive Myth
Uranium is not an explosive
In January, 1957, Walt Disney Studios released the animated short film Our Friend the Atom. It was an instant sensation, shown in theaters and classrooms across America. In it, a nuclear chain reaction was demonstrated using ping-pong balls and mousetraps. A large box was lined across the bottom with mousetraps loaded with two ping-pong balls each. One additional ball was tossed into the box, tripping one mousetrap, sending two other balls flying to trip other traps, and soon all the mousetraps were tripped with ping-pong balls flying everywhere. This was a very good demonstration of how the nuclear chain reaction worked for bombs, but it didn’t work for reactors. The reason is two-fold; first, the Uranium used in bombs is highly concentrated in Uranium isotope 235 (U-235) while reactors use only a very dilute, non-explosive concentration of U-235. Bomb grade Uranium (U-235) is so concentrated (>90%) that the high-energy neutrons released from each initial fission immediately causes just enough additional fissions to make the "prompt, supercritcal" explosion happen.
However, the neutrons from fresh fissions are about a million times too energetic to produce a chain reaction in low-concentration reactor fuel. This was either unknown to Disney’s staff, or considered too technical for the audience. Whatever the reason, the mousetrap and ping-pong ball demonstration resulted in misleading the public's understanding of chain reactions in bombs and reactors. They seemed to be the same. But, they are not.
It’s the wrong kind of Uranium
Naturally-occurring Uranium cannot be used to make a bomb because it is not a natural explosive. Natural Uranium is a uniform mix of two isotopes, U-238 and U-235. Natural Uranium is 99.3% U-238 and 0.7% U-235. U-238 is such a poor neutron-induced fissioner, under any conditions, that we can correctly say it won't experience a chain reaction in any way,shape, or form. It’s the U-235 that makes the nuclear chain reaction possible because it is a very good fissioner, relative to U-238. However, U-235 doesn't fission very much when bombarded by high energy neutrons which is the kind of neutrons released out of the fission.
In order to make a bomb core that will actually explode, the U-235 concentration must be increased to in excess of 90% to produce enough immediate fissions to make an explosion possible. This highly concentrated form of U-235 is necessary for a detonation...anything less won't work. This is in no way a secret, at least not any more. The U-235 concentration needed to make a bomb that works can be easily found in library encyclopedias and numerous websites on the internet. Regardless, anything less than a 90% U-235 concentration, and you can’t make a weapon small enough for a deliverable bomb…even if launched by a powerful rocket.
In theory, a ridiculously enormous amount of Uranium with about a 20% U-235 concentration is possible, but in no way realistic; the bomb would be bigger in diameter than the Empire State Building is tall. Less than a 20% concentration of U-235 and a nuclear explosion is absolutely impossible, no matter how much of the material is amassed. However, this is the reason that 20% "enriched" Uranium and Plutonium are defined as "weapon's grade". The 1-3% U-235, and/or Pu-239 in reactor fuels cannot explode, regarless of how much is amassed.
Power plant reactors never use Uranium with a high U-235 concentration, in order to keep fuel costs manageable. Back in the 1960s, early power plant reactors used Uranium with concentrations of U-235 in the 3-5% range. These were relatively small power plants using cores so small that a concentration increase of U-235 from the natural level was needed to sustain a chain reaction sufficient to produce electricity. As plants got bigger and the reactor cores larger, the U-235 concentrations dropped to between 1 and 3%. The precious few nuclear power plants completed in America after the Three Mile Island accident were quite large, and did not need the natural abundance of U-235 changed much at all. They used what is essentially natural Uranium, but those levels have been increased in order to allow longer in-core lifetimes between refuelings. In all cases, the concentration of U-235 found in any reactor fuel is way-too dilute to produce anything like a nuclear explosion. No matter how severe a reactor accident that can possibly be imagined, the fuel cannot explode like an atomic bomb.
How can an explosive be made out of something that is not itself an explosive? Perhaps the best commonly-known example is Nitrogen. About 79% of each breath you take is Nitrogen. No one would mistake it for an explosive. It is the wrong form of Nitrogen to detonate. However, chemically transformed from a gas into another, non-gasseous molecular structure, the Nitrogen becomes the primary active ingredient in Nitroglycerine and Tri-Nitro Toluene (TNT), which are unquestionably explosive. Devastating explosives can be made out of Nitrogen, which is not-itself an explosive. With Uranium, the natural form of the element must be metallurgically transformed into a highly un-natural type of Uranium in order to become the primary ingredient in a nuclear bomb. A terrible explosive can be made out of Uranium, which is not-itself an explosive.
Power plant reactors use a very dilute concentration of U-235 in their fuel, a level which can never cause a nuclear detonation. It’s not even weapon's grade. Power reactors cannot explode like a nuclear bomb.
Slowing down neutrons
As mentioned above, before freshly-released neutrons in a power plant reactor can cause fissions themselves, they must be de-energized. U-235 might not fission very often with high energy neutrons, but it fissions very well when engulfed in a field of low energy neutrons. To those in the nuclear sciences, it is described as "making thermal neutrons out of fast neutrons". The two technical terms for the process are thermalization and moderation. Freshly-released neutrons are roughly a million times too energetic to efficiently re-enter an un-fissioned U-235 atom, make its nucleus split (fission) and release 2 or 3 new neutrons (average of 2.5 per fission). To allow the chain reaction to proceed, the "fast" neutrons must be "slowed down" (moderated).
Four important points should be made. First, it is generally believed that the worst possible water-moderated reactor accident would be a sudden loss of all the water flowing through the reactor core. One thing is undeniable; without the water, there can be no chain reaction. A hypothetical complete-loss-of-water accident will completely stop the chain reaction itself. There are other severe issues to be confronted when this happens, but the reactor is totally shut down. (More on this in the page on TMI)
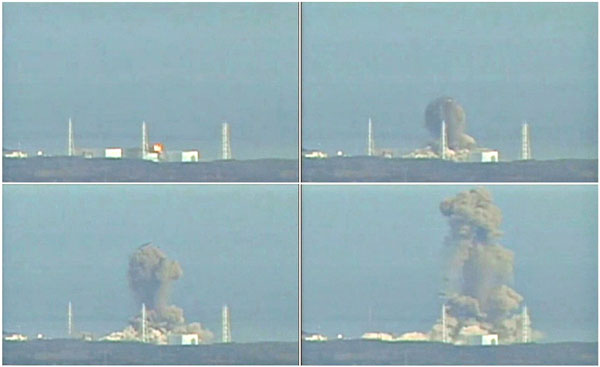
References:
- Langer, Mark; Disney’s Atomic Fleet; Animation World Magazine, Issue 3.1;
http://www.awn.com/mag/issue3.1/3.1pages/3.1langerdisney.html; April 1998 - Light Water Reactors; Hyperphysics; Department of Physics and Astronomy, Georgia State University; http://hyperphysics.phy-astr.gsu.edu/Hbase/nucene/ligwat.html#c1; 2005
- Montgomery, Jerry, PhD and Rondo, Jeffery, PhD; Asymmetrical Fission Products; Unclear to Nuclear; http://www.unclear2nuclear.com/asymFission.php; 2008
Next - Confusion about fallout https://www.hiroshimasyndrome.com/confusion-about-fallout.html